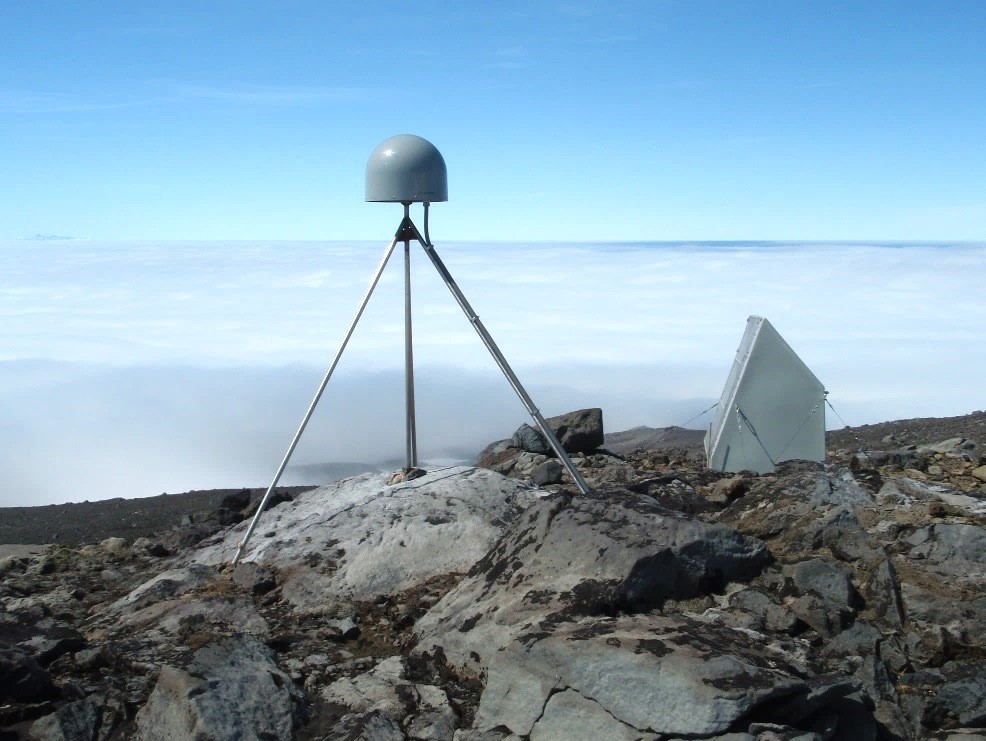
Global Navigation Satellite Systems (GNSS) such as Global Positioning Systems (GPS) consist of a number of satellites orbiting high above the Earth in near-circular orbits.
A receiver can determine its location to within a few metres by measuring the time it takes for a signal to travel to it from each satellite in view.
However, with the right equipment and careful data processing, centimetre-level positioning can be achieved. As such, GNSS is frequently used to measure many types of geophysical phenomena, such as the movement of tectonic plates of just a few millimetres per year, volcano inflation and deflation, and smaller-scale phenomena such as landslides.
GNSS can, for example, be used to determine the magnitude of large earthquakes. This is achieved by measuring the displacement of the ground caused by the earthquake itself at monitoring stations close to the fault.
For the largest earthquakes, such displacements can be huge: as large as several metres, and permanent displacements of a few millimetres can be identified thousands of kilometres away using GNSS.
Mathematical modelling can then be used to estimate the amount of slip along the fault itself. GNSS can be very beneficial in this application if the earthquake is very large. Such earthquakes are capable of causing devastating tsunamis, so a timely and accurate estimate of earthquake magnitude is crucial for any tsunami early warning system.
Seismometers are much more sensitive to movement, but cannot be relied upon to accurately estimate the magnitude of very large earthquakes because their measurements can literally go ‘off the scale’. In contrast, GNSS receivers do not suffer from this problem, although they are not as sensitive. The two technologies therefore complement each other well.
GNSS can also be used to measure the relatively slow deformation of the ground that occurs during the time between earthquake events. In plate boundary zones where most earthquakes occur, networks of GNSS monitoring stations can be used to estimate the amount of stress and strain on a fault and hence be used to estimate seismic hazard.
Similarly for volcano deformation: surface displacements measured by GNSS can be used to estimate the movement of magma deep underground. This information can help determine the likelihood of a volcanic eruption.
It is worth comparing GNSS with InSAR, which is widely used in the study of earthquakes and volcanoes. InSAR is capable of producing highly detailed satellite images showing any movement of the ground over a large area to a precision of just a few millimetres.
However, these displacements are measured in one dimension only: the direction in which the radar satellite is pointing. In contrast, GNSS can measure displacement in three dimensions, but only at specific points on the ground where the receivers are located.
Nevertheless, there are thousands of ground stations distributed around the world logging GNSS measurements continuously and these can often be accessed in real-time. If GNSS data is available, then it can be used to help ‘fill in the gaps’ between consecutive passes of InSAR satellites.
GNSS and InSAR are therefore complimentary to each other. Indeed, GNSS can also be used to measure the delay to signals from satellites as they pass through the troposphere, which can in turn be used to correct InSAR images.
In recent years, low-cost single-frequency ‘mass-market’ receiver modules and antennas have become available. These are small, lightweight and typically cost only a few tens of pounds each. With these components, it is possible to achieve similar levels of performance to geodetic-quality equipment but at a fraction of the price. This makes it feasible to deploy a network of several high-precision sensors without being precluded by cost.
This is an active research area in COMET. Such sensor networks could be used as part of a volcano or landslide monitoring system.
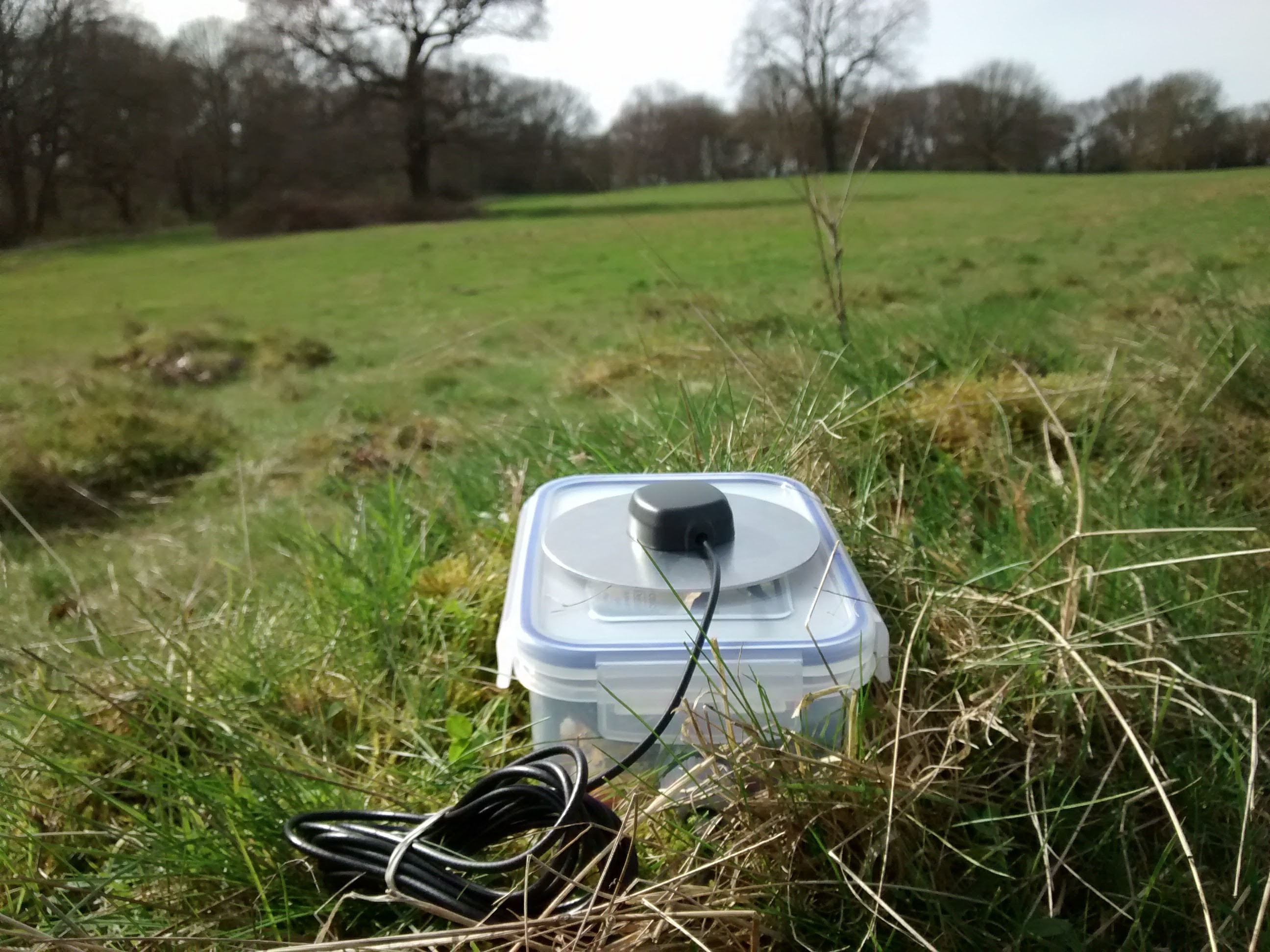
GNSS is therefore an effective tool for measuring geohazards and other geophysical phenomena in near real-time. However, data processing and interpretation must be handled carefully. Interference caused by the atmosphere and multipath (where GNSS signals get reflected off objects close to the receiver antenna) can introduce spurious position solutions.
Nevertheless, as the existing GPS and Russian GLONASS systems modernise, and with the addition of the European Galileo and Chinese BeiDou satellite navigation systems, further improvements in precision and accuracy are expected. Daily position estimates precise to the millimetre could enable new types of deformation to be identified.